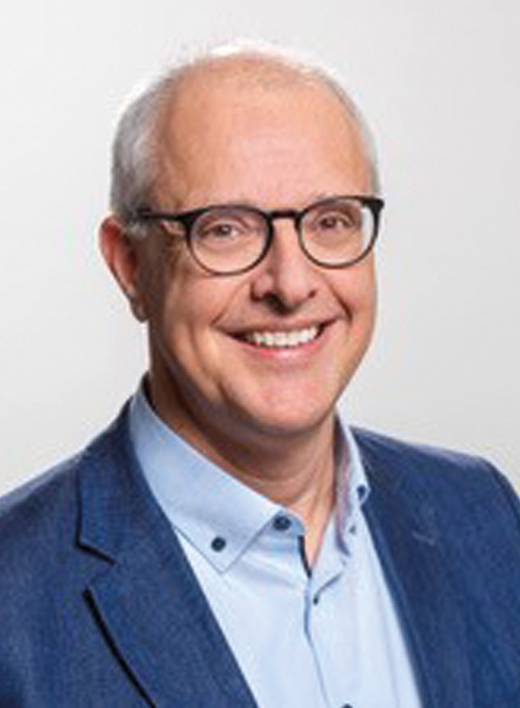
Andreas Bausch
Technische Universität München, Germany
Living matter relies on the self organization of its components into higher order structures, on the molecular as well as on the cellular, organ or even organism scale. Collective motion due to active transport processes has been shown to be a promising route for attributing fascinating order formation processes on these different length scales. Here I will present recent results on structure formation in organoid systems, demonstrating how mechanical feedback between extracellular matrix, proliferation and cell migration drives structure formation process in these multicellular model systems. I will present results on the developmental phase of mammary gland and pancreatic ducal adenocarcinoma organoids.
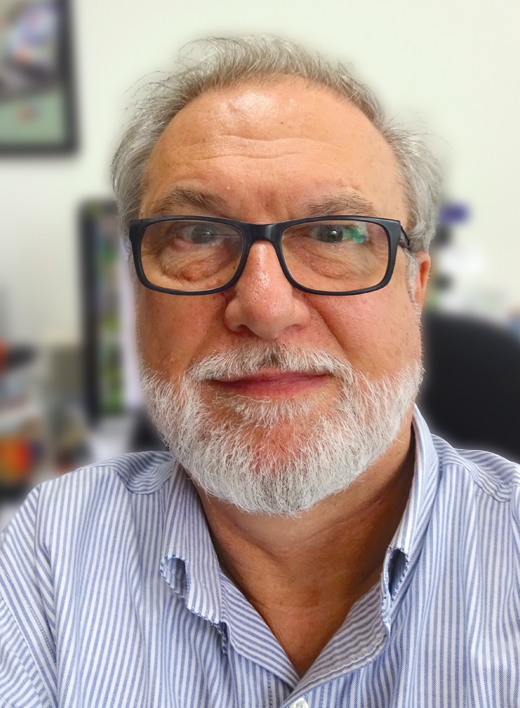
Hagan Bayley
University of Oxford, UK
By 3D printing, we have assembled synthetic tissues comprising patterned networks of thousands of aqueous droplets joined by lipid bilayers. A related printing technology has been used to pattern a variety of living cells, providing structures that include small tumours and fragments of neural tissue. The mm-scale printed structures can be used as building blocks for cm-scale structures ranging from synthetic axons to hybrid constructs containing both synthetic and living cells. An important goal is to be able to communicate with these constructs through external stimuli, have them process the incoming signals and accordingly produce outputs that are useful, for example, in medicine. Progress on these aspects of signaling will be described in my talk.
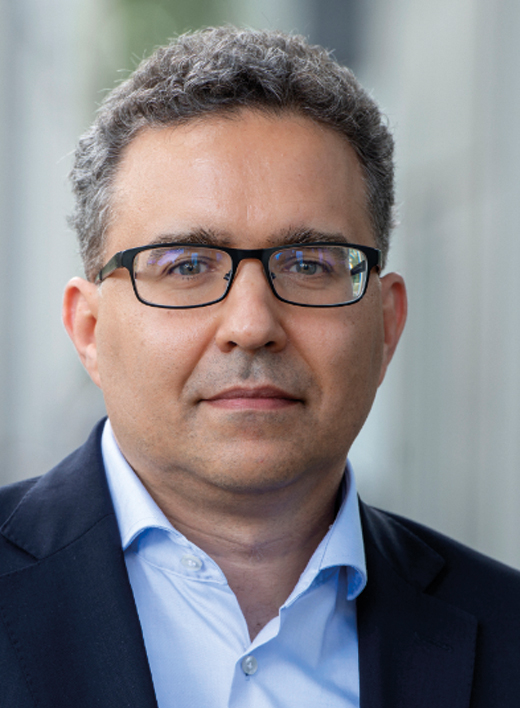
Michael Boutros
Heidelberg University, Germany
Patient-derived organoids are stem cell-derived 3D tumor models that can be efficiently established from cancer and normal tissues. The isolation of organoids from human primary tumors and metastases has enabled the establishment of living biobanks. We have performed image-based profiling using a high-throughput microscopy-based methodology to systematically measure phenotypes of in vitro models. When combined with chemical or genetic perturbations, image-based profiling can be a powerful approach to gain systematic insight into biological processes. However, performing large-scale image-based profiling experiments on organoids has been a biological, technical and computational challenge. As a result, the morphological heterogeneity of patient-derived cancer organoids between and within patient donors, their divergent behavior upon pharmacological perturbation, and the underlying mechanisms of cancer organoid morphology are not yet systematically understood. Here, we established a platform for large-scale image-based phenotyping of patient-derived cancer organoids to understand the underlying factors governing organoid morphology. Colorectal cancer organoids were treated with approximately 500 experimental and clinically used small molecules. We systematically mapped the morphological heterogeneity of organoids and their response to drug perturbations from more than 3,700,000 confocal microscopy images. We found that the resulting landscape of organoid phenotypes was driven by differences e.g. in organoid size, viability, and cystic vs. solid organoid architecture. We show that induced changes in morphology can be used to cluster small molecules by mechanism of action and infer the biological programs they affect.
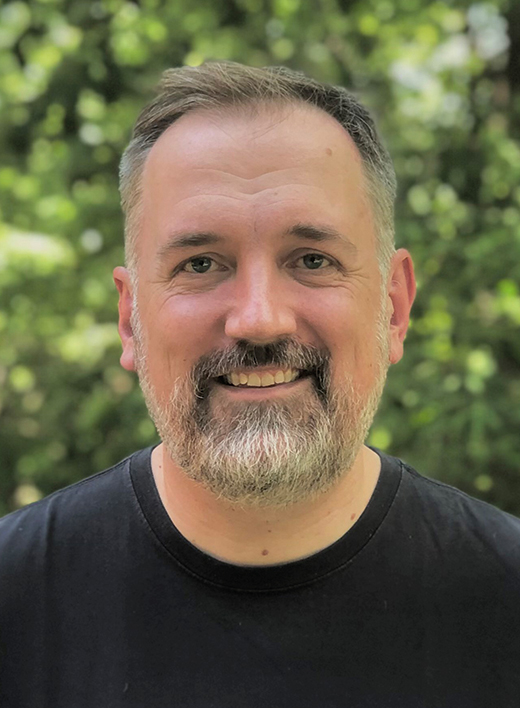
Jason Burdick
University of Colorado, USA
Cellular aggregates or spheroids are useful to understand basic biological processes or to act as building blocks in the engineering of tissues. Biomaterials are being used to advance spheroids in these applications, such as to guide their fusion or to control their organization. Our laboratory is particularly interested in understanding how engineered hydrogels can be used to support spheroids for use in regenerative medicine or as in vitro models of tissues.
In this presentation, I will give several examples of hydrogel technology to guide spheroid behavior. As a first example, we are using shear-yielding suspension baths to support the printing and fusion of spheroids into 3-dimensional tissues, where the bath properties guide print resolution and the stability of the printed structures. Next, hydrogel microparticles are being mixed with spheroids to act as living and non-living granular composites that are shear-thinning for injectability and where inter-particle microgel crosslinking stabilizes the granular structure for development into cartilage tissues. Lastly, by introducing degradation to the microgels, controlled contractility of the composites is possible, including with patterned structures where local contractility guides macroscopic shape evolution.
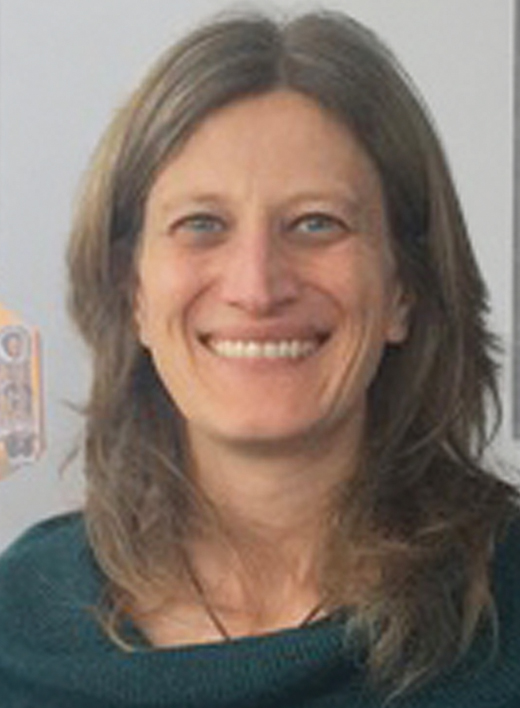
Ada Cavalcanti-Adam
University of Bayreuth, Germany
Cancer progression is a complex process involving a series of intricate cellular interactions and migrations. Tumor cell spheroids, three-dimensional aggregates of cancer cells, closely mimic the in vivo microenvironment and offer invaluable insights into the mechanisms of cancer invasion. In this talk, I will elucidate how different materials can steer the invasion patterns and the solid-to-fluid transitions of cancer cells. By utilizing state-of-the-art bioengineering techniques, we have created innovative in vitro models that accurately emulate the tumor microenvironment’s physical and biochemical cues.
Our findings demonstrate that specific material properties, such as stiffness, topography, and chemical composition, significantly impact the migratory behavior of cancer cell spheroids. Through advanced live-cell imaging and traction force microscopy, we decipher the intricate interplay between cancer cells and the surrounding material. By employing biofunctionalized materials and targeted drugs, we influence cancer cell behavior, potentially halting their invasive patterns and interfering with their collective responses to the microenvironment.
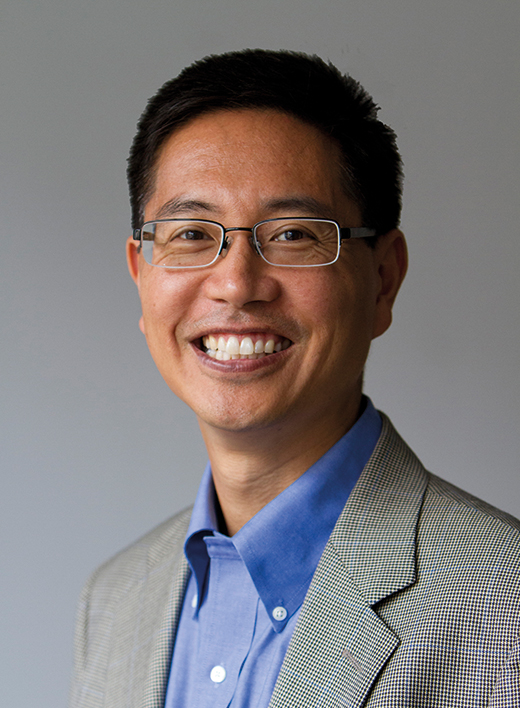
Christopher Chen
Boston University, USA
Mammalian tissues operate as highly integrated systems that link physical structure and biological function. We are attempting to rebuild tissue-like structures in vitro as a platform to better understand human physiology and disease, and as a stepping stone toward engineering tissues as therapeutics. Here, I will describe our recent work in engineering cardiac and vascular structures to understand the complex interplay between forces, signaling, and cellular adhesions in regulating tissue formation, function, and dysfunction. The presentation will highlight the importance of advancing numerous fabrication approaches to improve our ability to build tissues with ever increasing fidelity and function, and ultimately, how a deeper understanding of the interaction between tissue architectures and biological functionality will provide avenues to build better tools.
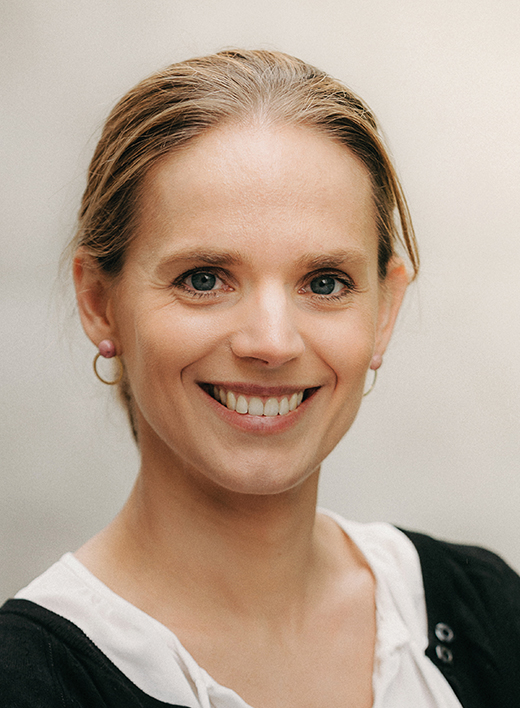
Laura De Laporte
RWTH Aachen University, Germany
We apply polymeric molecular and nano- to micron-scale building blocks to assemble into soft 3D biomaterials with anisotropic and dynamic properties. Microgels and fibers are produced by technologies based on fiber spinning, microfluidics, and in-mold polymerization. To arrange the building blocks in a spatially controlled manner, self-assembly mechanisms and alignment by external magnetic fields are employed. Reactive rod-shaped microgels interlink and form macroporous constructs supporting 3D cell growth or cells are able to use microgels as bricks to build their own house. On the other hand, the Anisogel technology offers a solution to regenerate sensitive tissues with an oriented architecture, which requires a low invasive therapy. It can be injected as a liquid and structured in situ in a controlled manner with defined biochemical, mechanical, and structural parameters. Magnetoceptive, anisometric microgels or short fibers are incorporated to create a unidirectional structure. Cells and nerves grow in a linear manner and the fibronectin produced by fibroblasts is aligned. Regenerated nerves are functional with spontaneous activity and electrical signals propagating along the anisotropy axis of the material. Another developed platform is a thermoresponsive hydrogel system, encapsulated with plasmonic gold-nanorods, which actuates by oscillating light. This system elucidates how rapid hydrogel beating affects cell migration, focal adhesions, native production of extracellular matrix, and nuclear translocation of mechanosensitive proteins, depending on the amplitude and frequency of actuation. The time spent in the in vitro gym seems to affect myoblast differentiation and fibrosis, while actuation seems to induce mesenchymal stem cell differentiation into bone cells.
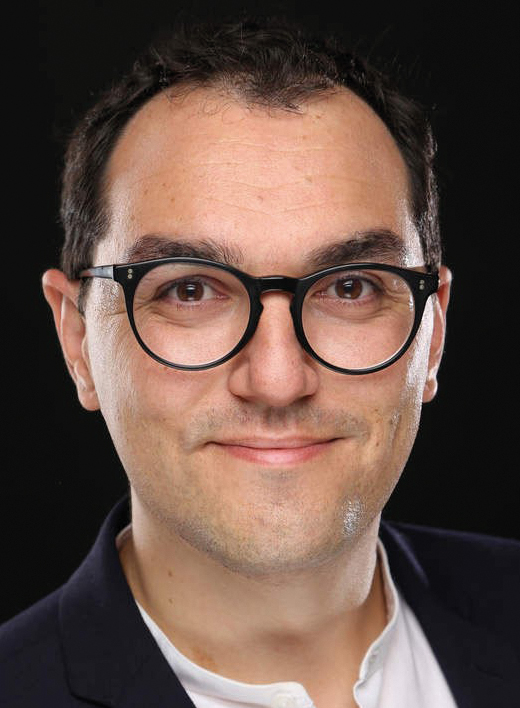
Jacopo di Russo
RWTH Aachen University, Germany
Mechanical properties regulate tissue functions at a multicellular length scale or mesoscale. These properties depend on the interaction of cells with their interfaces, hence on the balance between intercellular tension and the extracellular matrix (ECM) adhesion forces.
My group aims to dissect the role of cell-ECM and cell-cell communication in epithelial mechanobiology, starting from the medically relevant retinal epithelium. In contrast to the experimental investigation of traditional biological sciences, my laboratory uses cross-disciplinary approaches combining synthetic hydrogels with stem cell-based models. We particularly develop and adapt biohybrid systems where cells interact with hydrogels that are designed to control cell-cell or cell-ECM adhesion. Synthetic material allows the unique reduction of the degree of freedom in the cellular/tissue system, thus helping us to reveal phenotypical tissue plasticity and molecular function.
My talk will first give an overview of published work[1],[2] on understanding how ECM physical (elasticity) and biochemical cues (receptor density) impact epithelial system properties, namely stress heterogeneity and intercellular force coordination. I will show that these properties are not only in vitro observations but play pivotal roles in controlling our vision. A density gradient of ECM characterises the contractility of the retinal epithelium in vivo and modulates its efficiency in supporting photoreceptor cells’ homeostasis. Furthermore, I will show data from the ongoing work which addresses different aspects of the mechanobiology of tissue ageing. We optimised a phototunable hydrogel as substrates for epithelia to model ECM local remodelling on demand. Moreover, we developed microgels used as phototunable phantom cells to simulate age-related tissue mechanical anisotropy. Altogether, we can dissect the relationship between tissue mechanics and function by controlling the temporal and spatial properties of cellular interfaces.
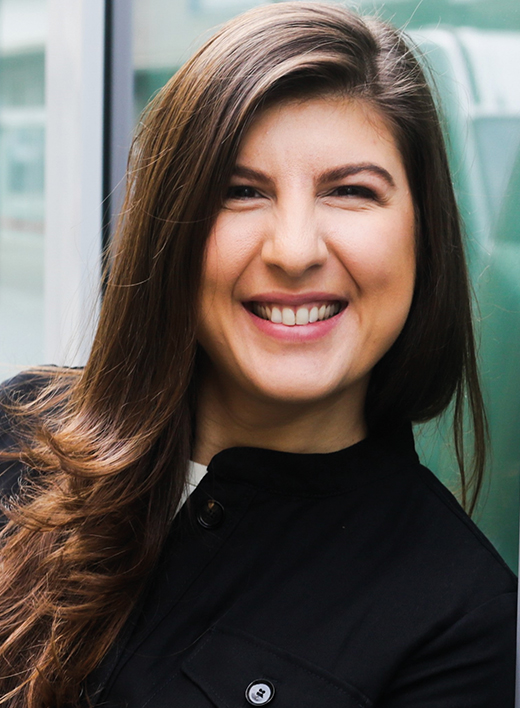
Daniela Duarte Campos
Heidelberg University, Germany
Bioprinting is an exciting technology that holds promise to fabricate tissues and organs both in vitro and in vivo. Besides engineering lab-grown in vitro models, current advances are focussed in bringing bioprinting technologies from the bench to the operating room. Scientists around the world are continuously improving the complexity and precision of bioprinting methods, which still in this decade will not only allow us to engineer new tissues and even organs, but also to change the way transplantation medicine is done. As with many new fields, this technology comes with challenges, such as controlling cell survival and function for being exposed to shear stress during bioprinting. This is one major hurdle that needs to be addressed by scientists in order to allow this young research field to achieve its first significant impact in medicine. In this talk, I will show bioinspired strategies and robotics that our lab is developing to minimize or flank the effect of shear stress for the purpose of building tissue using cells and cell aggregates.
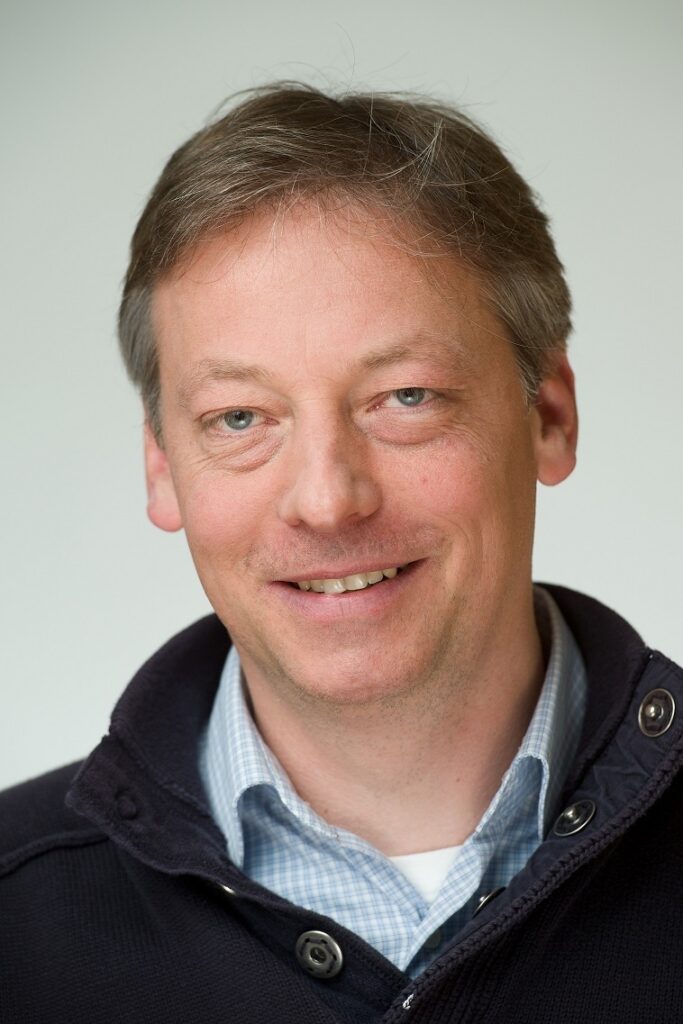
Peer Fischer
Heidelberg University, Germany
Is it possible to realize an assembler that can assemble biological cells into a defined shape of a 3D object, including cell aggregates and possibly organoids? We have recently developed tools that permit ultrasound fields to be shaped, such that they can be used to form defined pressure distributions in space with which it is possible to assemble cells from a suspension. In order to realize a 3D assembler, one needs to solve a formidable inverse problem: how to encode an entire 3D object in the form of sound waves that in turn are used to recreate the object in space. This is a complex optimization problem, because of the astronomically large number of computational degrees of freedom. Nevertheless, we have found practical solutions and we have managed to use the 3D pressure distributions to assemble cells in ‘one shot’. An acoustic holographic bioassembly platform will be presented and it is discussed how it can aid biofabrication and tissue engineering. We have also worked on a new type of ultrasound contrast agent – the antibubble – which is a microdoplet of fluid surrounded by air and whose release can be triggered by ultrasound. Antibubbles are remarkably stable and, importantly, their cargo can be released with low intensity ultrasound fields. They offer significant advantages compared with conventional ultrasound contrast agents and offer new ways to interact with cells and tissues.
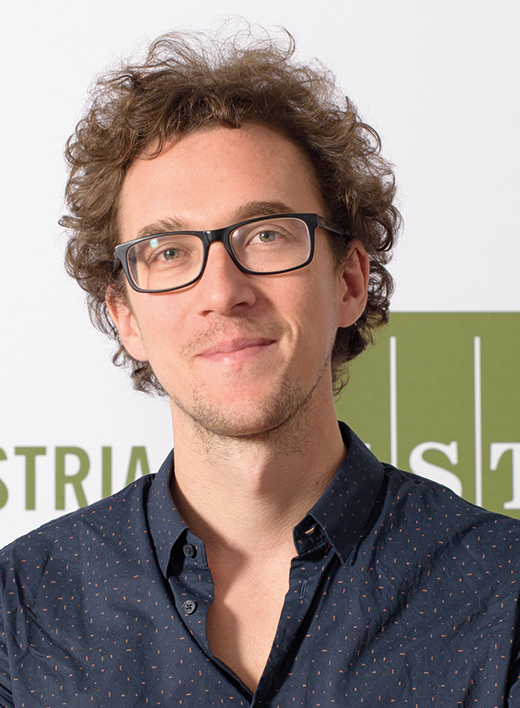
Edouard Hannezo
Institute of Science and Technology, Austria
How pattern and form are generated in a reproducible manner during embryogenesis remains poorly understood. Intestinal organoid morphogenesis involves a number of mechanochemical regulators, including cell-type specific cytoskeletal forces and osmotically-driven lumen volume changes. However, whether and how these forces are coordinated in time and space, via feedbacks, to ensure robust morphogenesis remains unclear. Here, we propose a minimal physical model of organoid morphogenesis with local cellular mechano-sensation, where lumen volume changes can impact epithelial shape, via both direct mechanical (passive) and indirect mechano-sensitive (active) mechanisms. We show how mechano-sensitive feedbacks on cytoskeletal tension generically give rise to morphological bistability, where both bulged (open) and budded (closed) crypt states are possible and dependent on the history of volume changes. We experimentally test key modelling assumptions via biophysical and pharmacological experiments, allowing us to quantitatively demonstrate how bistability can explain several paradoxical experimental observations, such as the importance of the timing of lumen shrinkage and robustness of the final morphogenetic state to mechanical perturbations. This suggests that bistability, arising from feedbacks between cellular tensions and fluid pressure, could be a general mechanism to coordinate multicellular shape changes in developing systems
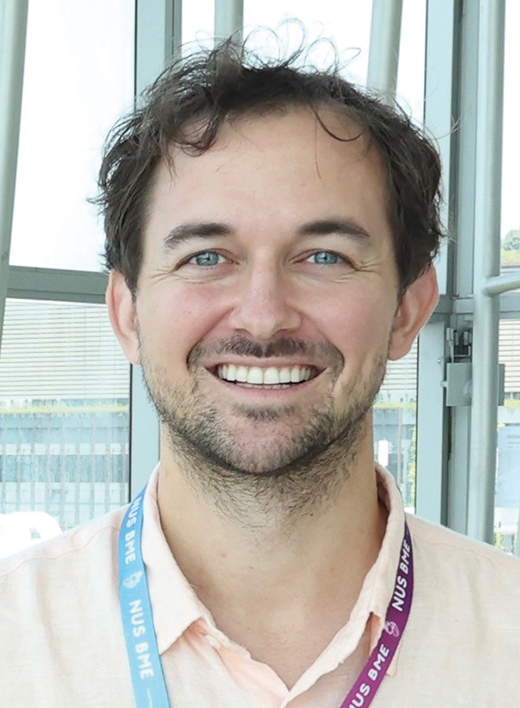
Andrew Holle
National University of Singapore, Singapore
Self-induced cellular confinement has been shown to play a role in a wide variety of biological processes, including cancer invasion and metastasis, immune cell navigation, and mechanosensitive gene expression and localization. Currently, the standard material-based approaches to presenting physiological confinement to cells, such as sandwich assays and microchannel chips, are frustratingly low-throughput and are not compatible with many emerging molecular biology approaches to elucidating cellular responses to their material microenvironment. To address this, we have developed a novel approach to presenting confinement to millions of cells in three dimensions that is also suitable for downstream molecular biology experimentation and future cellular engineering-based clinical applications. Inspired by a growing body of annealed granular hydrogel systems, we first constructed a granular system composed of glass microspheres with defined diameters. Due to gravity-induced spherical packing of glass microspheres, we can approximate the void space present in the system, as well as the confinement that cells are exposed to as they migrate within the material. As our granular system is not annealed, we can harvest cells growing within the material after long-term cell culture via trypsinization and gentle centrifugation. We found that cells grown in this granular material exhibit mechanical memory, as after harvesting their cell and nuclear morphology changes as a function of the dimensionality of the system. qPCR analysis of cells grown in granular materials with different diameter microspheres also reveals a modulation of gene expression as a function of void space and confinement, an experimental approach that was unavailable for conventional 3D confinement assays. Using this system, we have also found adult stem cells will preferentially differentiate towards an osteogenic lineage when presented with tighter physiological confining spaces. Future work from a materials perspective will focus on controlling the mechanical properties of the individual microspheres, applying systemic strain to the granular system, and building multi-component systems to encourage more complex cellular behaviour.
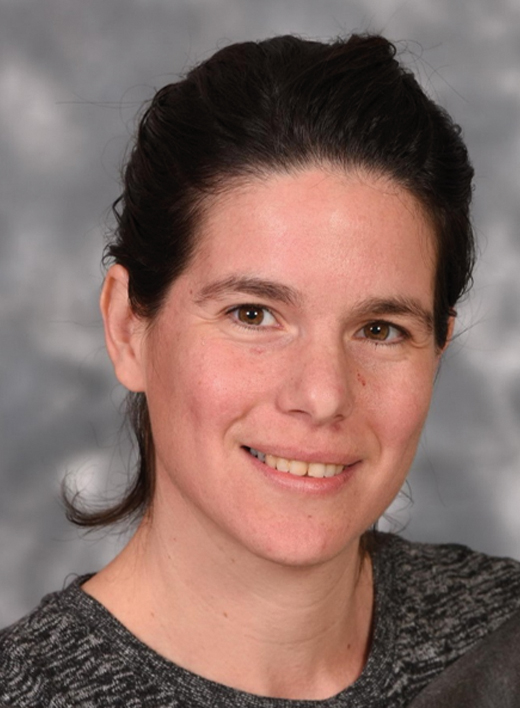
Ayelet Lesman
Tel Aviv University, Israel
University
The complex fibrous architecture of the extracellular matrix (ECM) facilitates the transmission of mechanical cues over long distances, allowing cells to mechanically interact with one another, even without direct physical contact. This intercellular interaction through the ECM can give rise to intriguing collaborative and collective cellular behaviors.
I will present our ongoing efforts aimed at unraveling the role of fibrous networks in mediating long-range cellular interactions. In our recent biological experiments, we embed cells in predetermined patterns, featuring controlled intercellular distances within 3D fibrous gels composed of collagen or fibrin. This approach enables both visual and quantitative assessments of the mechanical modifications within the ECM, shedding light on the relation between cell-induced mechanical remodeling and establishment of tissue-scale patterns and organizational states. To study the mechanical signals that guide cell behavior, we use optical tweezers microrheology to quantify the microscale mechanical properties of anisotropic and deformed fiber networks, reminiscent of the deformed ECM bands formed between contractile cells. Drawing inspiration rom the dynamic interplay between cells and their microenvironment, we engineer systems to guide and direct cell behaviour for potential applications in tissue engineering.
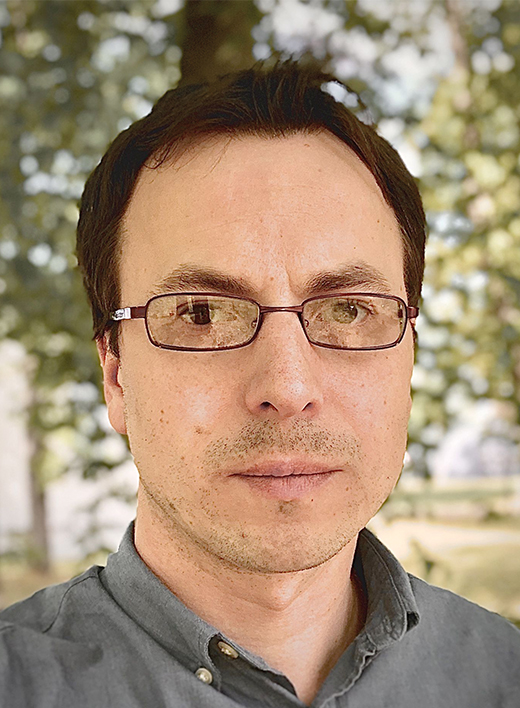
Pavel Levkin
Karlsruhe Institute of Technology (KIT), Germany
The fabrication of intricate 3D cell architectures is important in various fields including drug screening, tissue engineering, and 3D bioprinting. Despite its significance, achieving high-throughput fabrication of cell spheroids as well as the fabrication and manipulation of complex 3D cell architectures pose considerable challenges. In this presentation, I will present a method for fabricating complex multi-spheroid architectures in a high-throughput manner, using the droplet microarray platform. Our approach enables the formation of thousands of cell spheroids on a droplet microarray, which are subsequently assembled into multi-spheroid assembloids in an automated, controlled and high-throughput manner. This technique facilitates the formation of double, triple, and multi spheroids, each yielding distinct 3D architectures based on the cell type employed. Furthermore, we introduce a novel method for parallel high-throughput transfer of spheroids from one slide to another. In addition, a drug screening utilizing glioma cell spheroids formed on the chip and resulted in the identification of several promising drug candidates for the treatment of IDH1 mutant gliomas, will be presented. Finally, the utilization of cell spheroids as bioink additives, enabling their entrapment in 3D hydrogel structures formed using a novel parallel high-throughput 3D printing method will be shown. The future applications of complex cellular assembloids are vast and include personalized medicine, disease modeling, tissue engineering and regenerative medicine.
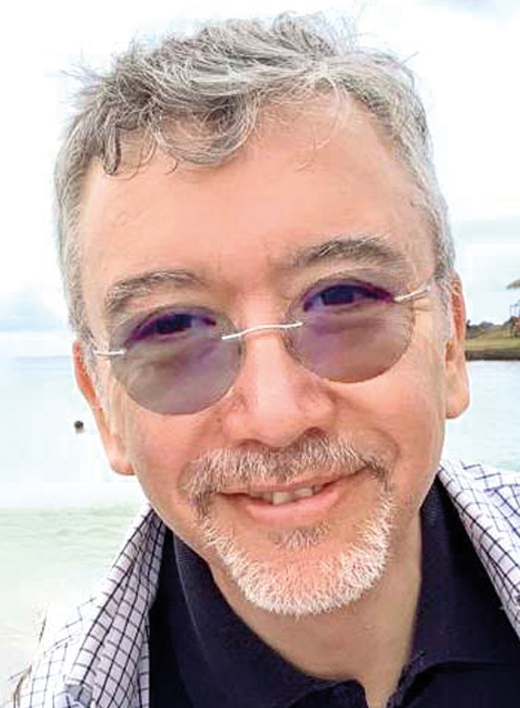
Roberto Mayor
University College London, UK
Although biomechanics is an active area of research, most of its studies are performed in vitro, ignoring the complexity of the 3D environment that cells encounter in vivo. We address this issue by analysing cell migration during embryo development, as it represents an excellent model for a complex 3D environment and, at the same time, is amenable to experimentation. We are interested in the mechanisms that control the initiation and directional migration of neural crest cells, a highly migratory and multipotent embryonic cell population whose behaviour has been likened to malignant invasion.
Our observations show that neural crest migration is triggered by an increase in the mechanical stiffness of the surrounding tissue. Once neural crest cells are migrating, they sense a combination of chemical (chemotaxis) and mechanical (durotaxis) cues that control their directionality. We conclude that neural crest cells are able to integrate chemical and mechanical cues to navigate in the complex 3D environment of the embryo.
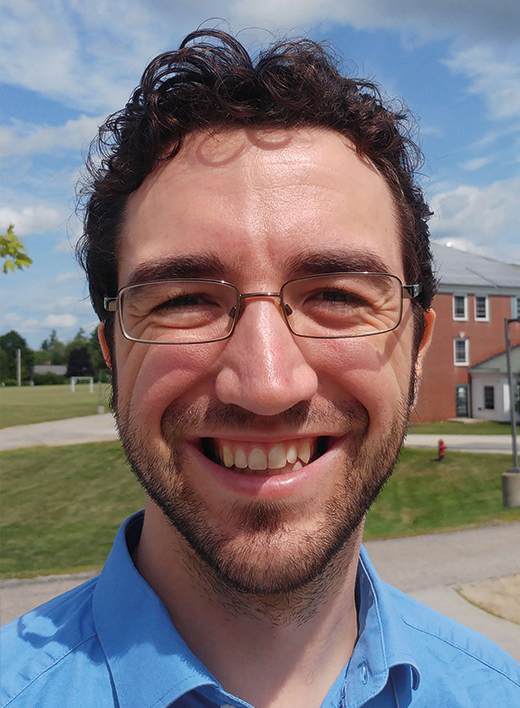
Matthias Merkel
Université Aix-Marseille, France
Most animals display one or more body axes (e.g. head-to-tail). In our work, we demonstrate that their formation can be promoted by large-scale tissue flows. We studied aggregates of mouse stem cells, called gastruloids, which are initially spherically symmetric, but later form an axis defined by the polarized expression of specific proteins. We show that advection of cells with tissue flows contribute substantially to the overall polarization, and that these flows are driven by interface and surface tension differences.
We further discuss flows in the context of anisotropic tissue deformation. From a physics perspective, anisotropically deforming tissues can be described as oriented active materials. However, such materials inherently exhibit instabilities, raising the question of how anisotropic tissue deformation during development can be robust. We show that the presence of a signaling gradient can stabilize the process, but only if it acts to actively extend the tissue along the gradient direction. Conversely, tissues are unstable if they tend to actively contract along the gradient direction. Intriguingly, developing tissues seem to exclusively use the gradient-extensile and not the unstable gradient-contractile coupling. Our work thus points to a principle of multi-cellular morphogenesis that is directly rooted in active matter physics, and which we expect to also affect multi-cellular engineering.
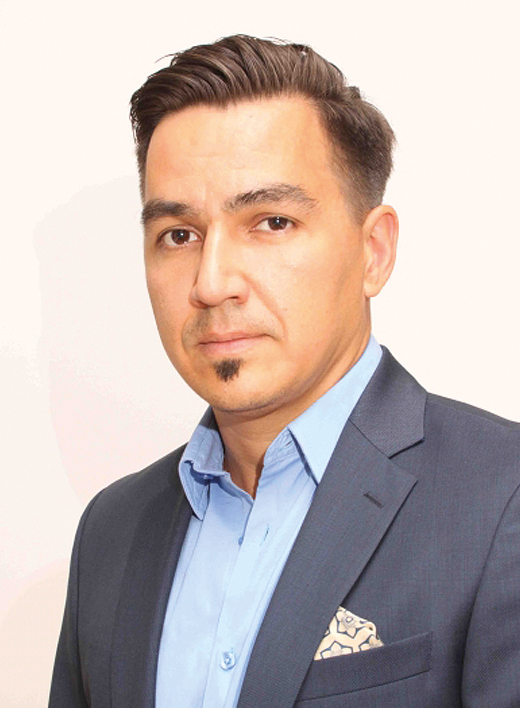
Aleksandr Ovsianikov
Technical University Wien, Austria
The most widespread 3D bioprinting technologies are based on computer-controlled deposition of cells or assembly of cellular units, and thus cannot achieve spatial resolution better than few tens of micrometres. Lithography-based methods approach the problem from a different direction, by producing 3D structures within cell-containing materials and can therefore overcome this limitation. Among these methods, multiphoton lithography (MPL) is an outstanding one as it can produce features even smaller than a single mammalian cell.
Our recent breakthroughs on the material development side enabled the use of MPL for direct fabrication of cell-containing constructs, placing this technology in the domain of high-definition (HD) bioprinting. In addition, we have recently demonstrated that MPL enabled realization of highly porous biodegradable microscaffolds capable of hosting individual cell spheroids. The resulting tissue units can be used for bottom-up self-assembly of larger tissue constructs with very high initial cell density, paving a way for novel tissue engineering approach.
In this contribution, the recent progress of MPL for biomedical applications, as well as its advantages and limitations will be discussed.
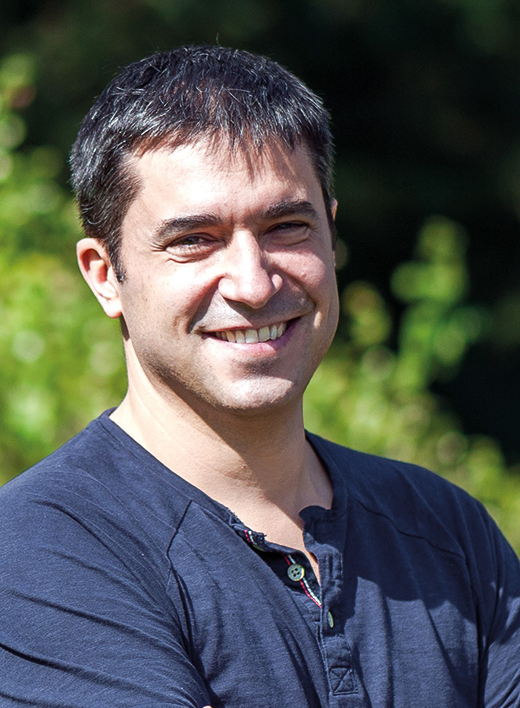
Aurélien Roux
Université de Genève, Switzerland
Liquid crystals are characterized by unique flow properties, dictated by the long-range order of molecules with anisotropic shapes that locally align at high density. Cellular tissues are viscoelastic materials, that have unique flow properties, best exemplified during morphogenesis: oriented flows participate in the formation of shapes and organs. Tissues are composed of cells, and cell shape and forces are dictated by contractile filaments of the cytoskeleton. Thus, both cell assemblies and cytoskeleton assemblies can be described as active nematics, which creates topological defects because of their activity (growth, contractility). In the small animal Hydra, the regeneration of head and foot correlates with the position of integer topological defects in the nematic field of actin, suggesting that topological defects in muscle cells could control or drive morphogenesis. I will further show that mechanical deformation of the nematic field of the actin in the Hydra causes bicephalous regeneration. Moreover, compressing the Hydra in different orientations, one can generate toroidal Hydra. When toroidal Hydra has no defects, as allowed by its unique topology, no head is regenerated, while toroidal Hydra with defects regenerate a head and a foot, keeping a hole in their body. Altogether, our findings support that topological defects in the nematic order of muscles cells or actin control the stress field in developing tissues, driving morphogenesis.
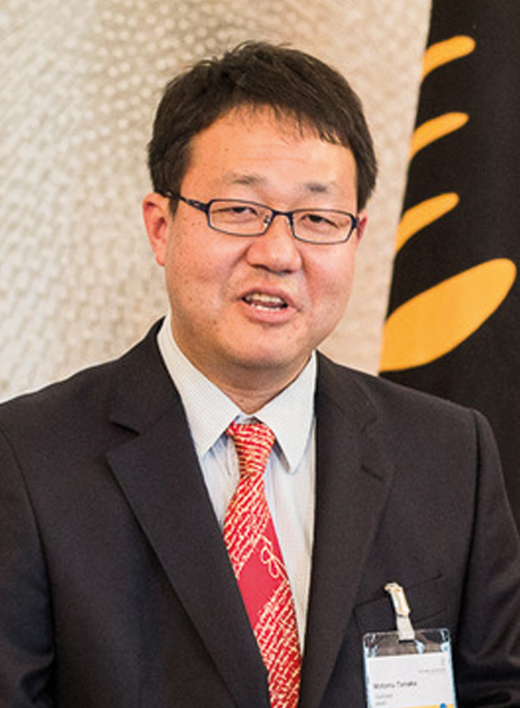
Motomu Tanaka
Heidelberg University, Germany
The formation of tissues and organoids includes many steps, e.g. lineage-specific differentiation of progenitors, cell sorting, and morphological adaptation. In addition to the biochemical cues like signaling, the tissue formation is driven physically, e.g. the mechanical interactions with extracellular matrix and neighboring cells and the intracellular contractile force.
In my talk, I am going to take two cases from our recent studies: (a) the role of collective cell orders in the maintenance of tissue homeostasis in human eyes [1], and (b) the interplay of intracellular forces and signaling during the symmetry breaking of Hydra tissues [2].
For the former, we established numerical indices that represent the spatial order of human corneal endothelium. From several quantitative indices, we found that the principle of colloidal physics helps us to predict the breakdown of the tissue homeostasis much earlier than the widely used diagnostic index. For the latter, we found the interplay of canonical Wnt signaling and dynamic deformation during the cell sorting and symmetry breaking in Hydra organoids.
Our data suggest that the well-defined 3D microenvironments could help us to guide the cell differentiation and pattern formation in organoids and tissues in vitro, which can contribute to develop new therapeutic tools.
[1] A. Yamamoto, H. Tanaka, M. Toda, C. Sotozono, J. Hamuro, S. Kinoshita, M. Ueno, M. Tanaka, A physical biomarker of the quality of cultured corneal endothelial cells and of the long-term prognosis of corneal restoration in patients, Nature Biomedical Engineering 3(12) (2019) 953-960.
[2] R. Suzuki, T. Hiraiwa, A. Tursch, S. Hoeger, K. Hayashi, S. Oezbek, T.W. Holstein, M. Tanaka, Spatio-temporal Coordination of Active Deformation Forces and Wnt/Hippo-Yap Signaling in Hydra Regeneration, bioRxiv (2023).
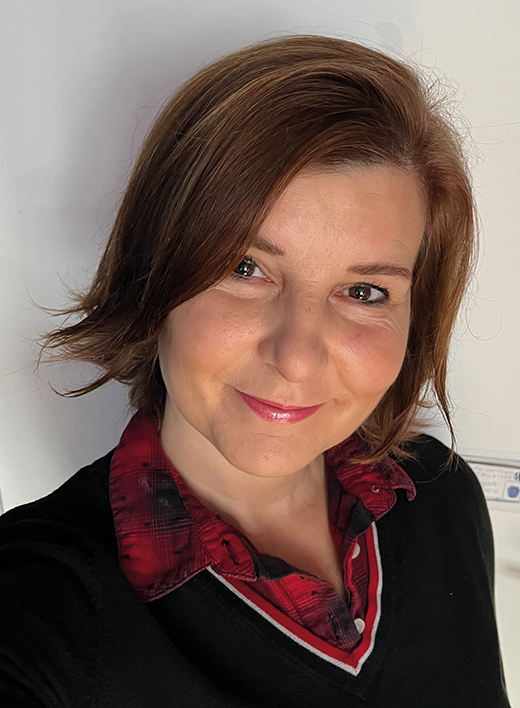
Danijela Vignjevic
Institut Curie Paris, France
The gut epithelium acts as a barrier between the outside world and the body while at the same time ensuring nutrient absorption. The small intestine epithelium comprises a single layer of columnar cells that line the villi that project into the gut’s lumen and the crypts that descend into the connective tissue. The constant epithelium renewal is achieved by stem cell proliferation in the crypts giving rise to specialized epithelial cell types. Upon exiting the crypt, most cell types migrate towards the villus tip, where they die and are shed into the lumen. The basal surface of the epithelium is underlined by the basement membrane, a thin and dense sheet-like structure on which cells adhere and migrate. While cells migrate collectively, maintaining their apicobasal polarity, they also display a second polarity axis (front-back), characterized by actin-rich basal protrusions oriented in the direction of migration. How this front-back polarity is established and what is the guidance cue for directional migration towards the tip of villi remains unknown. I will discuss our ongoing research aiming to address if and how the basement membrane provides cues for the directional migration of epithelial cells and what are the roles of adhesive structures in reading those cues. I will also discuss the role of the actomyosin cytoskeleton in maintaining intestinal epithelium integrity and the role of Piezo channels in the maintenance of the stem cell compartment. Finally, I will present our progress on Gut-on-Chip devices.
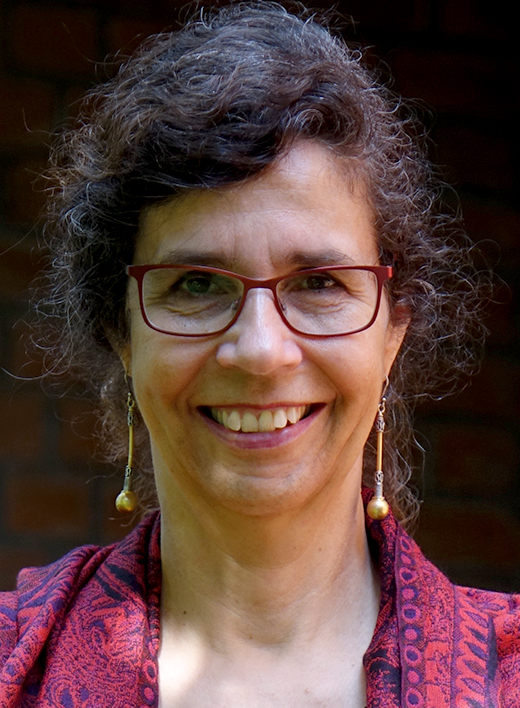
Viola Vogel
ETH Zürich Switzerland
While establishing that aggressiveness and malignancy of solid tumors correlates with tissue stiffening, the focus has been on deciphering the underlying mechanisms, which included quantifications of the Young’s moduli, the roles of enhanced interstitial pressure, changes in cell contractility, and extracellular matrix (ECM) remodeling and cross-linking. Yet, rather little is still known today how mechanical factors regulate cell fate in complex organ-specific cell niches. Progress is hampered as tissues are composed of a large variety of cell types which are in close contact to each other and communicate via biochemical and physical factors. Beyond the physical properties of cellular microenvironments, that engineers could easily produce and tune, the reciprocal mechanical signalling between cells and their extracellular matrix environments also involves the stretching of proteins and thus an off-on, or on-off switching of exposed molecular binding sites. Importantly, protein stretching can switch the structure-function relationships of extracellular as well as of intracellular proteins, which defines the underpinning structural principles of mechanobiology. Translating what has been learned in mechanobiology (mostly on single cells) to real organs, and finally to the clinic, was challenging due to the lack of nanoscale sensors to probe forces or tissue fiber tensions in healthy versus diseased organs. To bring our knowledge to the patient, we developed a nanosensor that probes the stretch-induced destruction of multivalent binding motifs, allowing us for the first time to visualize the tensional states proteins in animal models and in human tissues. Many unexpected findings were derived that were not anticipated from 2D cell culture studies. Underpinning mechano-regulated structural mechanisms and the significance of these findings in cancer and other pathologies will be discussed.
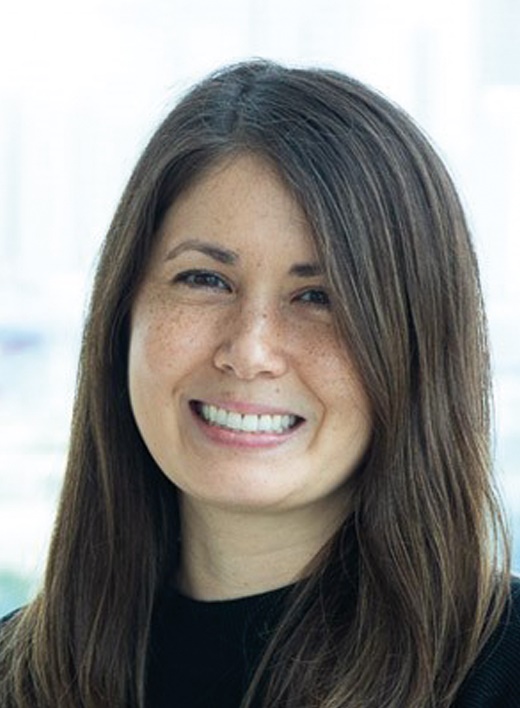
Jennifer Young
National University of Singapore, Singapore
It is well appreciated that extracellular cues stemming from the matrix dictate a multitude of cellular functions, from motility to stem cell differentiation. Yet, the extracellular environment is inherently complex and thus hinders our full understanding of specific matrix-based contributions to cellular behavior. Our work focuses on age-related extracellular matrix (ECM) remodeling and engineering materials capable of recapitulating ECM properties in vitro at both the micro and nano length scales. This talk will highlight some of our approaches in ECM tissue characterization and materials design to control cell-matrix interactions in the context of cardiac aging and mechanobiology. We describe a tunable stiffness gradient polyacrylamide (PA) hydrogel system using a two-step polymerization method capable of spanning the diverse physiological and pathological mechanical landscapes present in the heart (e.g., Young’s modulus, E ~10-50 kPa). We apply these stiffness gradient hydrogels to understand the regulation of mechanosensitive processes on cardiac function in an age-dependent manner. We also describe a new material platform consisting of tunable hybrid hydrogel-decellularized cardiac tissues that maintains age-specific native matrix composition and organization independent of matrix stiffness. Subsequent culture of cardiac fibroblasts (young and aged) show that the matrix ‘age’ can outweigh matrix mechanics in driving fibroblast activation. Our strategies ultimately aim to interrogate the cell-matrix interface using highly defined biomaterial systems at different length scales that can inform future matrix-based treatment strategies.