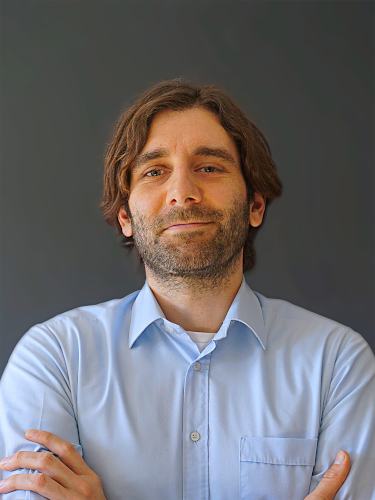
Jens Bauer
Karlsruhe Institute of Technology (KIT), Germany
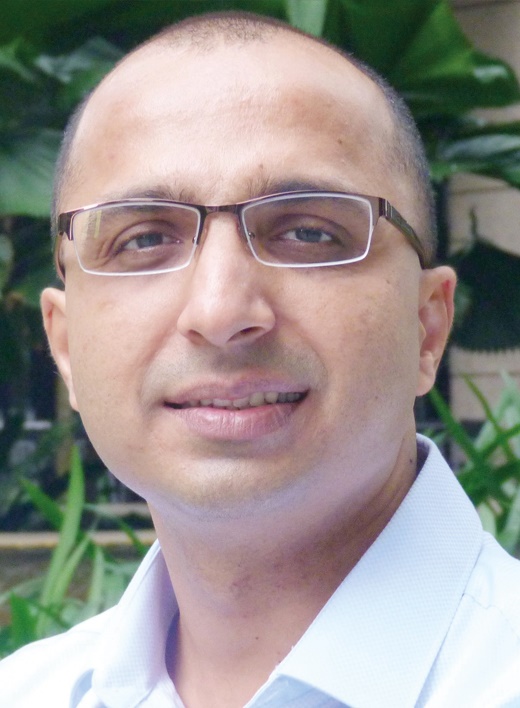
Akshay Bhinge
University of Exeter, UK
Amyotrophic lateral sclerosis (ALS) is a devastating neurodegenerative disease characterized by progressive motor neuron loss. Though animal models have provided key insights into disease pathogenesis, species-specific differences have hampered translation of these findings to humans. Human induced pluripotent stem cells (iPSCs) have revolutionized our ability to study ALS by providing patient-derived models that capture disease-relevant cellular phenotypes. Using CRISPR-Cas9, we have engineered iPSCs to create physiologically relevant human models that recapitulate key cellular, molecular and biochemical features observed in ALS patient neurons. Our models exhibit hallmark neurodegenerative phenotypes, offering a robust platform for mechanistic studies. We are now advancing these models into complex, multicellular 3D neural systems to better understand cell-cell interactions in disease, ultimately paving the way for therapeutic discovery.
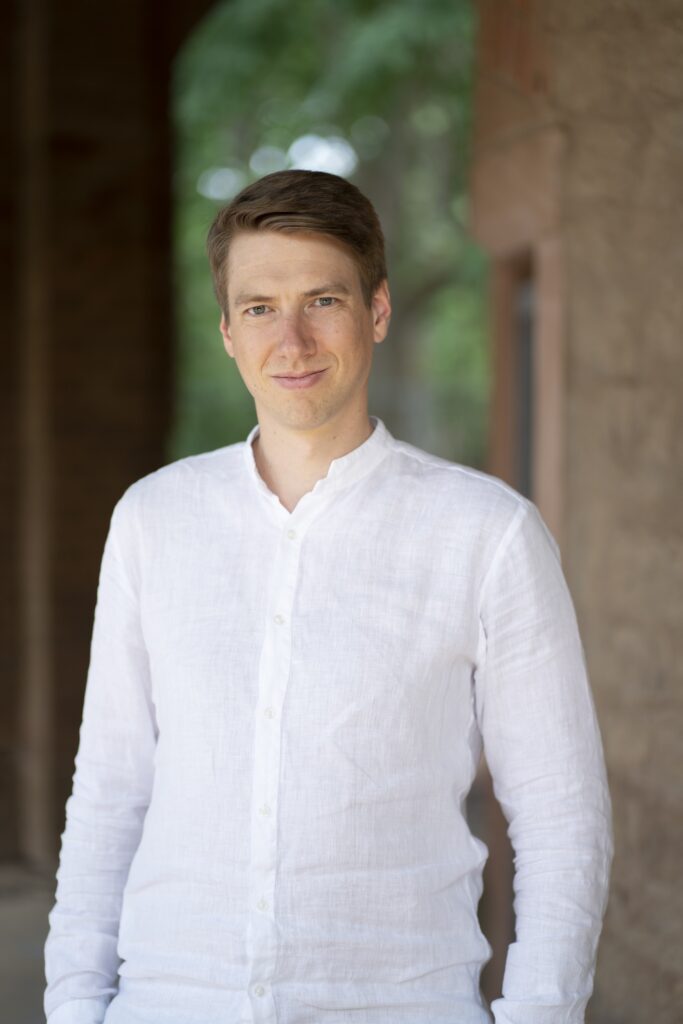
Simon Binder
Karlsruhe Institute of Technology (KIT), Germany
Hydrogels are a group of soft materials which show excellent biocompatibility but can also be designed to respond with a volume change to parameters such as glucose, salinity or complex biomarkers. This makes them attractive for sensing applications, in particular for medical devices and implants. The talk highlights how hydrogel-based sensors work and the various ways a hydrogel’s volume response can be transduced into an electrical signal. The sensors presented are intended for applications in drug monitoring or blood glucose measurement, both as point-of-care device or subcutaneous implant. Moreover, hydrogels may also serve as scaffolds for guided cell growth. This is being utilised in neural implants for the brain-computer-interface. Starting from the “Utah Array” neural implant, an insight into a biohybrid version of such an implant will be given. The biohybrid approach is expected to result in both improved implant acceptance as well as recording of neural activity with high spatial resolution.
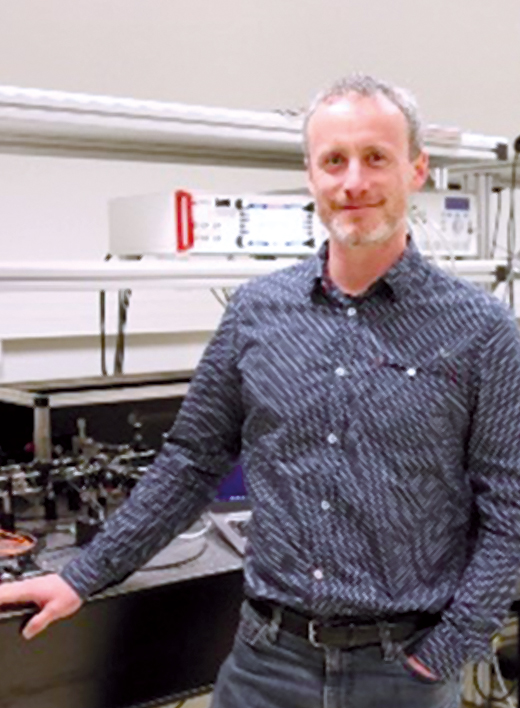
Daniel Brunner
Institut FEMO-ST, France
Neural network (NN) concepts revolutionize computing by solving challenges previously thought to be reserved to the abstract intelligence of humans. However, the astonishing and substantial conceptual breakthroughs are so far not mirrored by advances in integrated hardware specialized in physically implementing NNs. As always with computing, scalability is the key metric. Integrated photonic architectures have the potential to revolutionize energy consumption and speed. However, conventional 2D lithography strongly limits the size of integrated NNs due to fundamental scaling laws. We want to overcome this problem by using 3D printed photonic integration, where photonic waveguides realizing a NN’s connections.
Bio:
Daniel Brunner is a CNRS researcher with the FEMTO-ST, France. His interests include novel computing using quantum or nonlinear substrates with a focuses on photonic neural networks. He has received several University and the IOP’s 2010 Roys prize, the IOP Journal Of Physics: Photonics emerging leader 2021 prize as well as the CNRS Bronze medal in 2022. He edited one Book and two special issues, has presented his results 50+ times upon invitation, has published 70+ scientific articles, has been awarded a prestigious ERC Consolidator grant and is a pilot of the BEP-PEPR Electronique project of the France 2030 initiative.
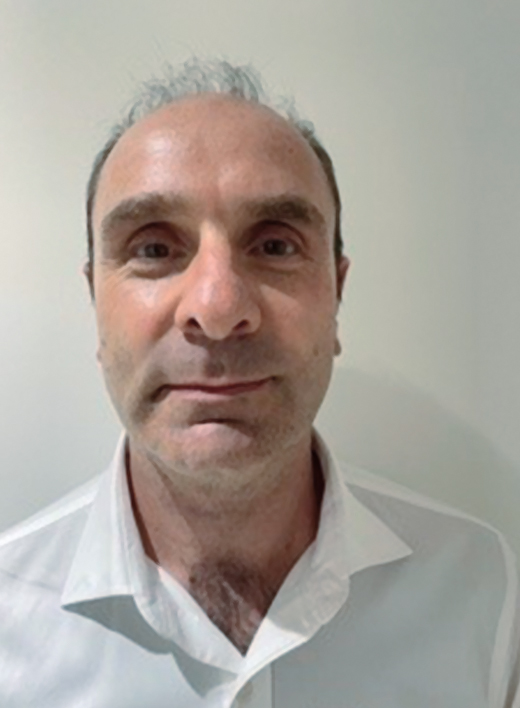
Fabio Cicoira
Polytechnique Montréal, Canada
Materials able to regenerate after damage have attracted a great deal of attention since the ancient times. For instance, self-healing concretes, able to resist earthquakes, aging, weather, and seawater are known since the times of ancient Rome and are still the object of research.
While several mechanically healable materials have been reported, self-healing conductors are still relatively rare, and are attracting enormous interest for applications in electronic skin, wearable and stretchable sensors, actuators, transistors, energy harvesting, and storage devices, such as batteries and supercapacitors.1 Self-healable and recyclable conducting materials have the potential to reduce electronic waste by enabling the repair and reuse of electronic components, which can extend the lifespan of electronic devices. Furthermore, they can be used for wearable electronic and biomedical devices, which are often subject to mechanical stress causing damage to their components.
Conducting polymers exhibit attractive properties that makes them ideal materials for bioelectronics and stretchable electronics, such as mixed ionic-electronic conductivity, leading to low interfacial impedance, tunability by chemical synthesis, ease of process via solution process and printing, and biomechanical compatibility with living tissues. However, they show typically poor mechanical properties and are therefore not suitable as self-healing materials.
In our group, we produced several self-healing and stretchable conductors by mixing aqueous suspensions of the conducting polymer poly(3,4-ethylenedioxythiophene) polystyrene sulfonate (PEDOT:PSS) with other materials providing the mechanical characteristics leading to self-healing, like for instance polyvinyl alcohol (PVA), polyethylene glycol, polyurethanes and tannic acid. 2-10 In this talk, various types of self-healing will be presented and correlated with the electrical and mechanical properties of the materials. The use of the self-healing gels and films as epidermal electrodes and other devices will be also discussed.
Conductive materials obtained from blends of polyurethane-PEDOT:PSS and PEG showcase exceptional stretchability, toughness, and self-healing properties. Moreover, these materials can be recycled several times and maintain their mechanical and electrical properties.
- Li, X. Zhou, B. Sarkar, F. Cicoira et al., Adv. Mater. 2108932, 2022.
- Li, X. Li, S. Zhang, F. Cicoira et al., Adv. Funct. Mater. 30, 2002853, 2020.
- Li, X. Li, R. N., S. Zhang, F. Cicoira, et al. Flexible and Printed Electronics 4, 044004, 2019.
- Zhang, Y. Li, F. Cicoira et al. Adv. Electron. Mater1900191, 2019.
- Zhang, F. Cicoira, Adv. Mater. 29, 1703098, 2017.
- Zhou, G. A. Lodygensky, F. Cicoira et al., Acta Biomaterialia139, 296-306, 2022.
- Kateb et al., Flexible and Printed Electronics, 8 (4), 045006, 2024.
- Zhou, F. Cicoira et al., J. Mater. Chem. C, 12, 5708, 2024.
- J. Kim., F. Cicoira et al. Mater. Horiz. 11, 348, 2024.
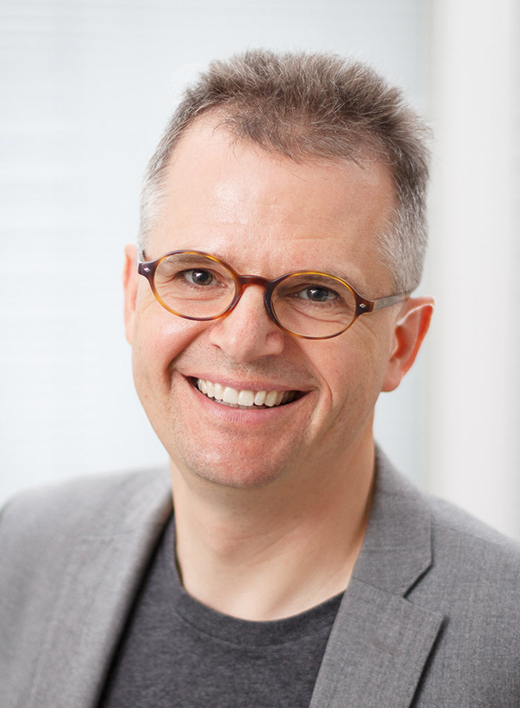
Harald Gießen
Stuttgart University, Germany
We utilize femtosecond 3D printing to generate complex 3D microoptics, consisting of aspherical systems which include also doublet or multiplett lenses.
We demonstrate achromatic systems using hybrid refractive/diffractive optics as well as achromats using different materials with varying refractive index and dispersion.
Wavefront measurements of the 3D printed systems indicate aberrations over the entire image field of less than lambda/10.
These systems can be printed directly onto single mode or multicore optical fibers, to realize ultrasmall endoscopes and side-looking OCT systems for blood vessels. We have demonstrated imaging of plaques in the coronary arteries of living pigs. When printed onto single quantum emitters or single photon detectors, they allow for efficient in- and out-coupling to single mode fibers for quantum technologies.
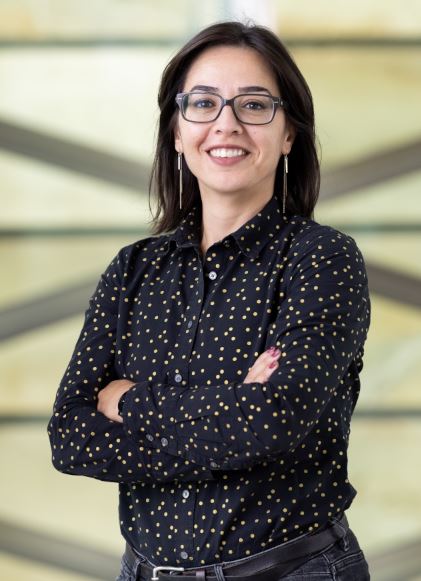
Sahika Inal
King Abdullah University of Science and Technology (KAUST), Saudi Arabia
Organic mixed ionic and electronic charge conductors offer a unique toolbox for establishing electrical communication with biological systems. In this talk, I will introduce this rising class of materials for bioelectronic interfacing and explain how their multifunctionality can be harnessed to develop next-generation optoelectronic devices operating at aqueous electrolyte interfaces. I will specifically highlight one application where these devices are used to detect biochemical molecules. I will discuss two types of organic electronic sensors: one designed to detect Alzheimer’s disease-associated proteins, surpassing the performance of current state-of-the-art methods, and another capable of detecting coronavirus spike proteins at the physical limit. Drawing from our experience with patient samples, I will address potential shortcomings of proof-of-concept biosensor platforms and explore strategies for overcoming these challenges. By tackling these problems, we improve device performance to a level that marks a considerable step toward biochemical sensing of infectious and noninfectious disease biomarkers.
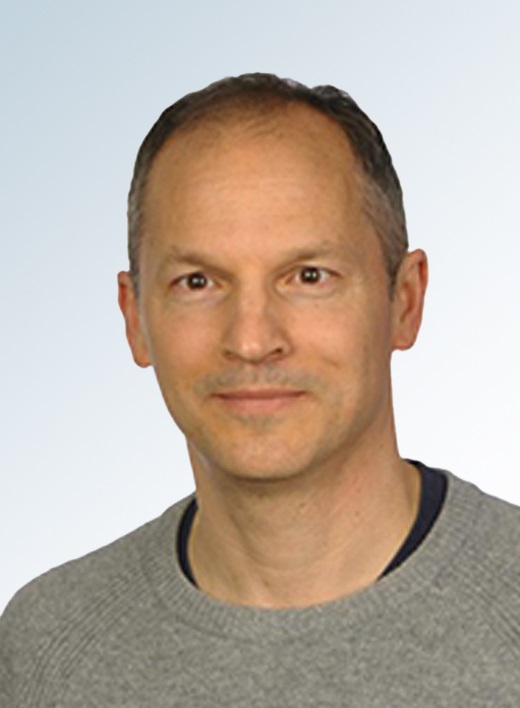
Gaspar Jékely
Heidelberg University, Germany
Neuronal connectomics aims to map synaptic wiring diagrams in blocks of neural tissue or across entire organisms. Currently, only electron microscopy can provide synaptic resolution, but other modalities such as coherent X-ray and expansion microscopy are rapidly catching up. I will talk about our volume EM pipeline and how we use it to map whole-body neural circuits in small aquatic animals. In the experimentally accessible and small larval stages of the marine annelid Platynereis, we can combine whole-body connectomics with neuronal activity imaging, behavioural experiments, transgenesis and CRISPR manipulations, to link genes to neurons and behaviours. Similar approaches could be used to characterise structure-function relationships in neuronal organoids or hybrid neuron-on-chip systems.
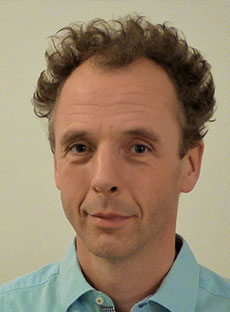
Martijn Kemerink
Heidelberg University, Germany
The sheer infinite freedom to design and synthesize organic molecules allows, amongst many other things, to fuse multiple functionalities in a single compound. In this talk I will focus on a specific class of small molecular materials that combine dipolar and semiconducting functionalities. When brought into a (solid) state with sufficient long-range order, dipole-dipole interaction can give rise to a ferroelectric state. It turns out that the ferroelectric polarization couples to the charge transport, leading to a conductivity that is different for current flow parallel and anti-parallel to the polarization direction. Since typical ferroelectric materials show (meta) stable intermediate polarization states, the resulting material has a continuously tunable conductivity, which might be relevant for neuromorphic applications. In this talk, I will present the current state of the art in terms of materials and formal understanding.
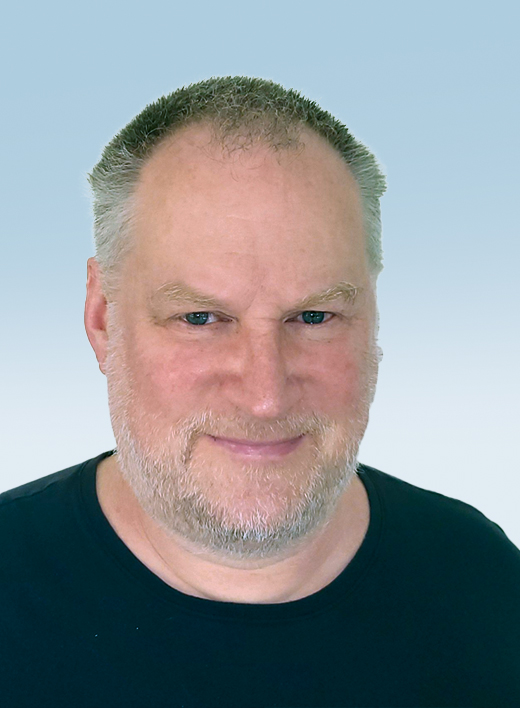
Jürgen Klingauf
University of Münster, Germany
In the central nervous system communication between neurons occurs at specialized junctions called synapses, whereby a single neuron may be in contact with many other neurons by hundreds of synapses. At the chemical synapse, electrical signals trigger controlled secretion of neurotransmitter via exocytosis of synaptic vesicles at the presynaptic site. The neurotransmitters diffuse across the synaptic cleft and activate postsynaptic receptor channels, generating an electric signal in the postsynaptic cell. Progress in identifying the molecular mechanisms of synaptic vesicle fusion and recycling, however, has been hampered by the use of mass culturing systems which do not allow quantitative assessment at the individual synapse level and due to the small size of presynaptic boutons close to the diffraction limit of light. We developed a purely presynaptic preparation, the xenapse, presynaptic boutons formed by cultured mouse hippocampal neurons or by induced human neurons on micropatterned host substrates (glass coverslips or chips) functionalized with synaptogenic cell adhesion proteins. Xenapses are formed en face directly onto the glass substrate enabling optical single vesicle recording by total internal reflection microscopy. Xenapses show all the hallmarks of a typical synapse both structurally and physiologically. In summary, we established a more homogenous and scalable synaptic culture system with robust synapse formation, that can be used to interface living synapses with nanostructured biomimetic surfaces and communicate with single synaptic contacts in a biohybrid system.
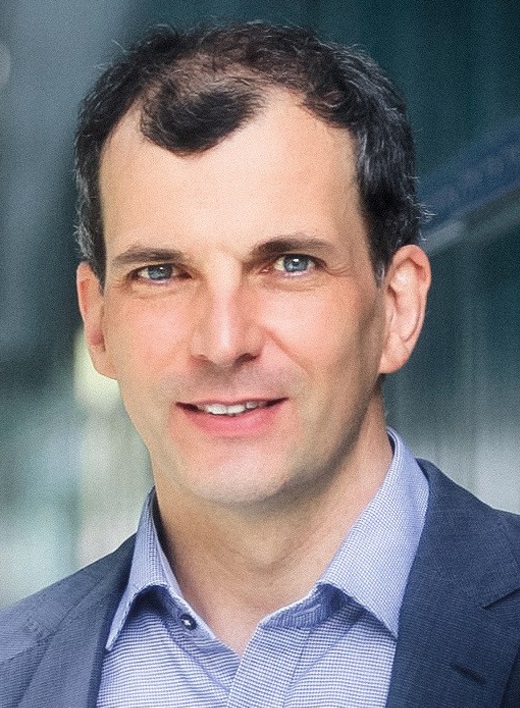
Moritz Kreysing
Karlsruhe Institute of Technology (KIT), Germany
Optical traps have long been proposed for the precision assembly of microstructures, but their utility is limited by the material properties of both the medium and the particles.
Here, as an alternative, we employ laser-generated thermoviscous flows to achieve precise alignment of micro-objects regardless of their material characteristics. By creating complex laminar flow fields, we demonstrate micro-robotics with up to 30 degrees of freedom in viscous solutions.
Formulated as a global and fully differentiable optimization problem, our model-based approach nowyields (i) increased robustness and convergence and (ii) emergent collective behavior, and (iii) paves the way toward AI-controlled, objective-oriented micromanipulations.
Current research involves integrating 3D-printed channels to adavance thermoviscous flows manipulation within confined spaces, enhance imaging capabilities, and broaden the possibilities for manipulating biological cells.
Our results establish high-definition microfluidic manipulations with transformative potential for assembly, augmented micro-manufacturing, micro-robotics and optically actuated micro-factories, where the right things happen to be at the right place at the right time.
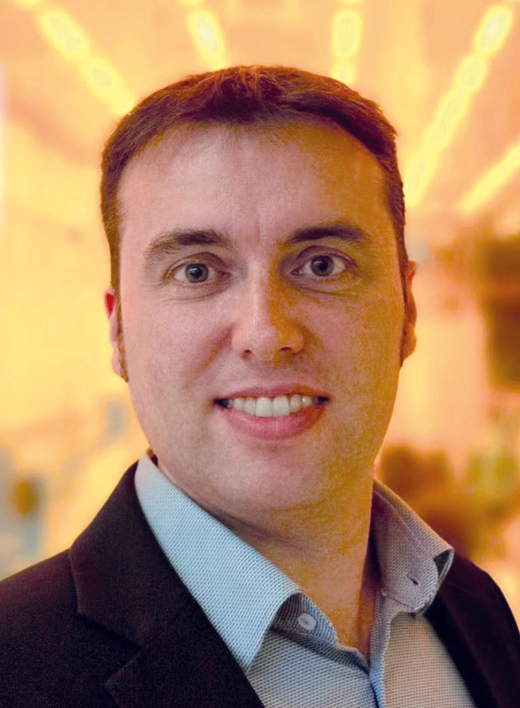
Mario Lanza
National University of Singapore
Abstract
Two-dimensional (2D) materials have outstanding physical, chemical and thermal properties that make them attractive for the fabrication of solid-state micro/nano-electronic devices and circuits. However, synthesizing high-quality 2D materials at the wafer scale is difficult, and integrating them in silicon microchips brings associated multiple challenges. Nevertheless, in the past few years substantial progress has been achieved and leading companies like TSMC, Intel and Samsung have started to work in this direction too. In this talk I will discuss how to integrate 2D materials in micro/nano-electronic devices, circuits, and microchips, giving a general overview of the global progress achieved in the field and presenting our last developments in hybrid 2D/CMOS applications. I will put special emphasis on devices and circuits for memristive technologies, including data storage, computation, encryption, and communication. I will also discuss the main technological challenges to face in the next years and provide some recommendations on how to solve them.
Biography
Mario Lanza is an Associate Professor of Materials Science and Engineering at the National University of Singapore. His research focuses on improving electronic devices, integrated circuits, and silicon microchips using novel nanomaterials. He has published over 200 research articles in top journals including Nature, Science and Nature Electronics, and he has been plenary, keynote, tutorial and invited speaker in over 150 conferences. For this work, he and his students have received some of the most prestigious awards in the world (such as the IEEE Fellow) and his articles have been highly cited. He is often consulted by leading semiconductor companies and top publishers. He is an active member of the board of governors of the IEEE – Electron Devices Society, and has been involved in the technical and management committee of top conferences in the field of micro/nano electronics, including IEDM and IRPS.
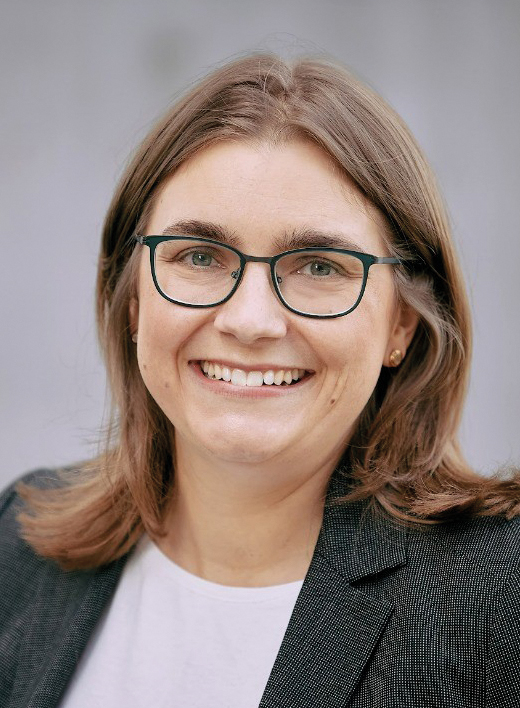
Simone Mayer
Karlsruhe Institute of Technology (KIT), Germany
The human brain is characterized by unique features that are not present in other species including animal models. The development of brain organoids derived from pluripotent stem cells over the last decade has revolutionized our understanding of human brain development at the molecular and cellular level. Simone Mayer is using brain organoids as a tool to model diverse neurodevelopmental and neurodegenerative disorders caused by genetic and environmental impacts. Specifically for the rare pediatric disorder pontocerebellar hypoplasia, Simone Mayer has recently established brain region-specific organoid models that recapitulate the pathology in the neocortex and cerebellum. Using this unique tool, she is now investigating disease mechanisms as well as developing therapeutic approaches. Additionally, the group is working on developing organoids as a model system further by enhancing analysis methods as well as through bioengineering approaches.
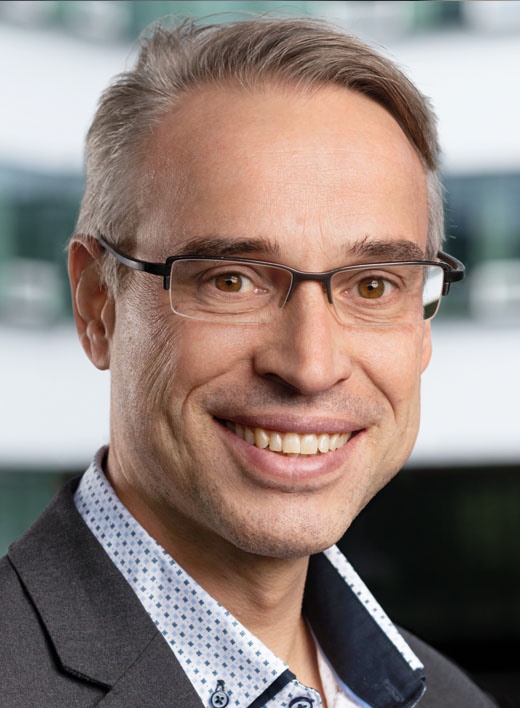
Christian Nijhuis
University of Twente, The Netherlands
Our brains constitute a molecular computer that is able to process enormous amounts of information with a tiny energy budget [1]. Inspired by the energy efficiency of brains and the ever-increasing demand for miniaturised electronics, there is a drive to develop devices that mimic the dynamic character of neurons and synapses. To achieve this goal, brain-like computing is emulated with energy inefficient and complex silicon-based circuits or with mesoscale memristors, but these approaches still require large amounts of energy. For these reasons, it is important to develop new types of hardware that can mimic brain-like computation processes [1,2]. We have been developing molecular switches that behave like synapses with the aim to realize spiking neural networks. I will introduce a new type of molecular switch that can remember its switching history [3]. By coupling fast electron transport to slow proton addition steps via dynamic covalent bonds, the switches display time-dependent switching probabilities which can be used for brains-inspired and reconfigurable electronics [4,5,6]. These artificial synapses are promising to develop alternative neural networks and open new ways to design electronic devices by exploiting their inherent dynamical properties.
References
- Christensen et al. Comput. Eng. 2022, 2, 022501.
- Zhu, J., Zhang, T., Yang, Y.; Huang, R. A Phys. Rev. 2020, 7, 011312.
- Thompson, D.; Barco, E. d.; Nijhuis, C. A. Phys. Lett. 117, 030502 (2020).
- Wang, Y.; Zhang, Q.; Nickle, C.; Venkatakrishnarao, D.; Zhang, Z.; Leoncini A.; Qi, D.-C.; Han, Y.; del Barco, E.; Thompson, D.; Nijhuis, C. A. Mater. 2022, 21, 1403-14011.
- Zhang, Q.; Wang, Y.; Nickle, C.; Zhang, Z.; Leonchini, A.; Qi, D.-C.; Sotthewes, k.; Borrini, A.; Zandvliet, H.J.W.; del Barco, E.; Thompson, D.; Nijhuis, C. A. Commun. 2024, 15, 8300.
- Wang, Y.; Zhang, Q.; Nickle, C.; Zhang, Z.; Leonchini, A.; Qi, D.-C.; Borrini, A.; Han, Y,; del Barco, E.; Thompson, D.; Nijhuis, C. A. Nanoscale Horiz., 2025: DOI: 10.1039/D4NH00211C
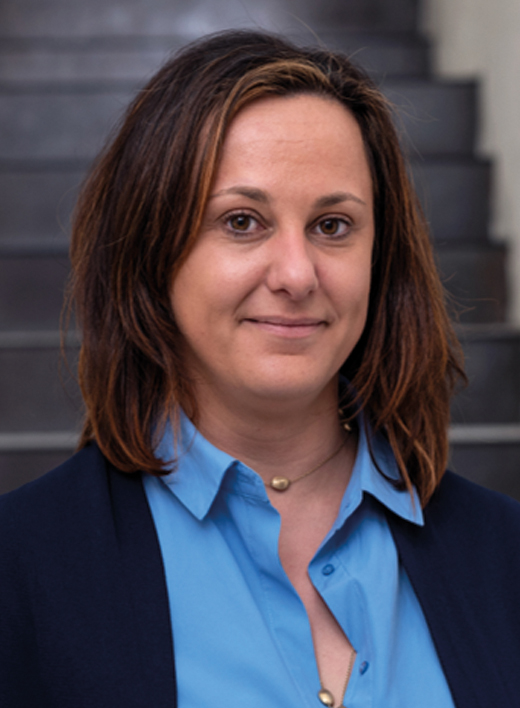
Luisa Petti
Free University of Bozen-Bolzano, Italy
Recent advances in materials science and manufacturing have enhanced electronic devices by adding features like flexibility, stretchability, biocompatibility, and biodegradability. These capabilities are essential for smart devices that adapt to 3D surfaces, dissolve in specific environments, and interact with biological systems, benefiting fields such as environmental monitoring, wearable technology, medical implants, and sustainable agriculture. Biopolymers, as renewable and eco-friendly materials, provide a promising foundation for these technologies while addressing concerns about electronic waste.
Nanocellulose (CNC) and chitin nanocrystals (ChNC) are notable biopolymers due to their biodegradability and sustainable production. CNC offers high mechanical strength, optical transparency, and good dielectric properties, while chitosan, derived from chitin, is abundant, biocompatible, and possesses anti-bacterial and bio-adhesive properties. Demonstrated applications include flexible resistive temperature detectors (RTDs) and thermistors on chitosan substrates, which can be used to monitor body fluids, pollutants, and plant health, providing sustainable bio-interfacing options.
Biopolymer electrolytes also hold promise for solid-state organic electrochemical transistors (OECTs), which use electrolytes to conduct ions within an organic mixed ionic-electronic conductor. Unlike traditional electrolytes, biopolymer-based versions are biodegradable and derived from renewable resources, helping reduce environmental impact. Here we show printed chitosan- and agar-based electrolytes in OECTs enabling applications like biosensing, wearable technology, and neuromorphic circuits.
In addition to electronics, biopolymers act as scaffolds for cell growth and plant support in biohybrid systems. Hydrogels like polyethylene oxide (PEO) and alginate, used in 3D bioprinting, support cell growth and tissue regeneration. In agriculture, superabsorbent biopolymer scaffolds aid plant growth and water retention, with minimal environmental impact. This integration of biopolymers, electronics, and living systems can drive sustainable next-generation devices, aligning technology with nature.
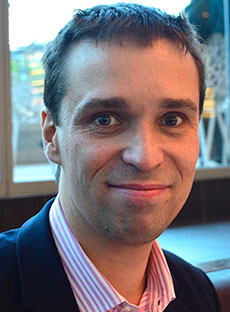
Carsten Rockstuhl
Karlsruhe Institute of Technology (KIT), Germany
Nowadays, we can define the spatial distribution of materials over many centimeters with a typical voxel size well below one micrometer thanks to 3D nanoprinting technology. The materials in reach with such a technology offer unprecedented degrees of freedom to control light propagation. In this contribution, we outline various approaches to provide digital blueprints for structured photonic materials that are feasible for fabrication with additive manufacturing techniques and tailored to meet demands in various applications. The structures we design comprise complex free-form surfaces and fully structured photonic materials in 3D. Multiple approaches for solving the inverse problem are exploited in this endeavor, e.g., the adjoint method, automatic differentiation, and machine learning-based methods.
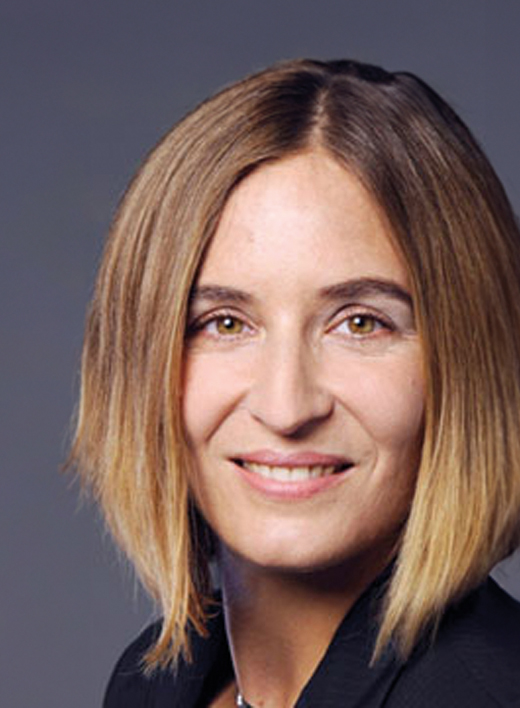
Ruth Schwaiger
Forschungszentrum Jülich GmbH, Germany
Carbon-based materials, ranging from amorphous carbon to nanostructured graphene and
diamond-like carbon, exhibit exceptional mechanical properties such as high strength, elasticity,
and wear resistance. These properties, combined with their chemical stability and lightweight
nature, make them ideal for diverse applications in optics, electronics, and bioengineering. The
ability to fine-tune the microstructure through advanced additive fabrication techniques allows
unprecedented control over their mechanical behavior and functional performance.
This presentation will explore the intersection of additive nano- and micro-fabrication and carbonbased
materials, focusing on how microstructural engineering influences mechanical properties.
We will discuss key fabrication techniques, which enable precise structural control at the
nanoscale. Furthermore, we will highlight how these innovations translate to real-world
applications underscoring the extraordinary potential of carbon-based materials in pushing the
boundaries of next-generation technologies.
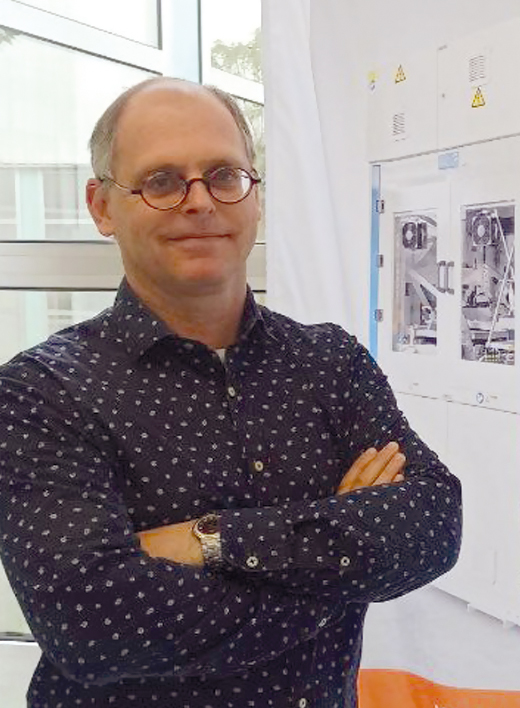
Marc Verschuuren
Rob Voorkamp
SCIL Nanoimprint Solutions, The Netherlands
Nanoimprint is a relatively new form of lithography where a patterned stamp is used to replicate the information. Substrate Conformal Imprint Lithography (SCIL) uses a soft-stamp based NIL technique and still achieves high resolution, low pattern deformation, and sub-micron overlay alignment.
Most NIL techniques use organic imprint resists that cure (transform from liquid to a solid after moulding into the inverse stamp shape) due to a crosslinking reaction initiated by UV radiation. Although being versatile, these organic materials do have disadvantages related to material stability under (blue) light and at elevated temperatures.
Inorganic materials made through a sol-gel route offer an inorganic based crosslinking route towards the direct replication of full inorganic patterns, offering additional functionality (chemical, physical). By optimizing the resist and imprint system together, a moderate shrinkage of ~ 8% has been achieved in combination with room temperature pattern formation within 1 minute. The resist systems cover a refractive index as low as 1.18 up to 2.1 in the visible and is UV and visible light stable and can retain 30nm patterns up to 1100°C. Faithful replication of patterns down to sub-10nm, aspect ratios >6 and pattern reproducibility with less than 1nm variation over 300mm wafers have been demonstrated. Additionally, the silicone rubber based stamps allow demoulding of negative release pattern, such as slanted gratings.
In the contribution, we will show examples of materials used for nano-photonics (meta-surfaces, DOEs), laser, bio-applications and possibilities for electronic components.
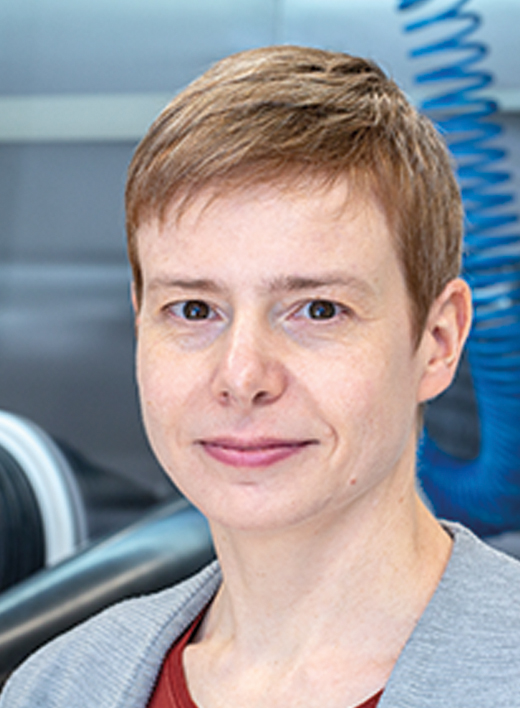
Jana Zaumseil
Heidelberg University, Germany
Advanced dispersion and sorting methods have enabled the purification of large amounts of semiconducting single-walled carbon nanotubes (SWCNTs) with different diameters and bandgaps that can be integrated in various (opto-)electronic devices with outstanding properties. For example, field-effect and electrochemical transistors based on printed networks of sorted SWCNTs exhibit high charge carrier mobilities (>10 cm²/(Vs)) and on/off current ratios (> 108) that are suitable for circuits or sensors. Chemically doped SWCNT films show high electrical conductivities, high Seebeck coefficients as well as excellent power factors (up to 920 µW m-1 K-2) that make them a highly promising material for flexible thermoelectric generators. To further improve performance, it is important to understand the interplay of a multitude of parameters such as nanotube length, diameter distribution, alignment, n- and p-doping, dielectric environment, intentional and unintentional defects and their impact on macroscopic charge transport. Moreover, the controlled introduction of luminescent defects through covalent functionalization leads to substantially enhanced near-infrared photo[1]luminescence for bio-imaging, optical sensors, light-emitting devices and even single[1]photon emitters, which opens up an even wider range of applications for these unique one-dimensional and printable semiconductors. I will give an overview of our recent results and insights on covalent functionalization of SWCNTs, the impact of surface passivation on device performance and how to achieve stable p-doping via pH[1]dependent proton-coupled electron transfer.